News
Study Reveals Slow Stress Release as Key to Earthquake Ignition
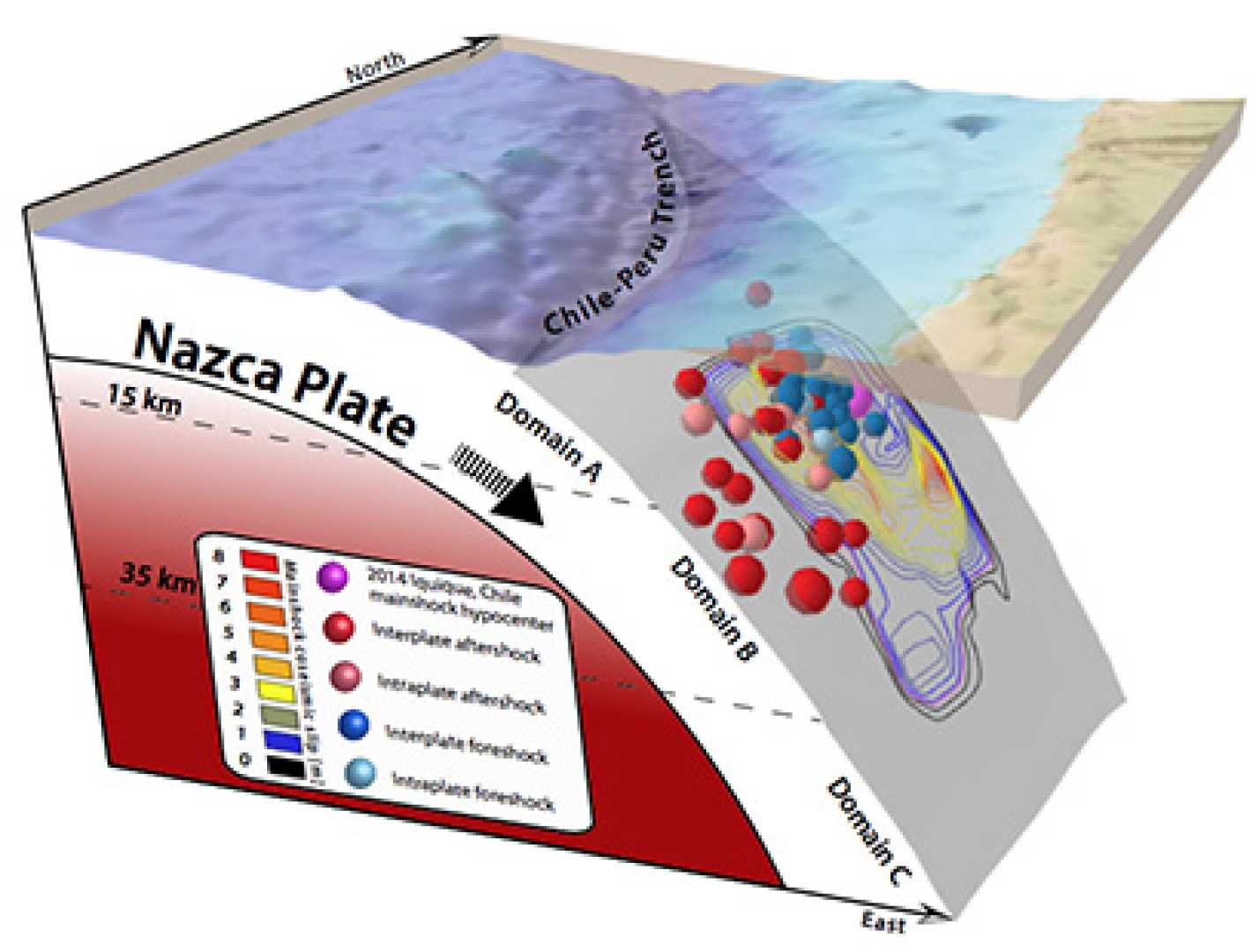
A groundbreaking study published in Nature on January 8, 2025, has uncovered the hidden mechanics behind earthquake ignition, revealing that slow, silent stress release plays a critical role in triggering seismic activity. Conducted by researchers from the Racah Institute of Physics at the Hebrew University of Jerusalem, ETH Zurich, and École Normale Supérieure de Lyon, the study challenges traditional models of earthquake nucleation and offers new insights into the transition from creeping motion to violent ruptures.
Led by Prof. Jay Fineberg and Ph.D. student Shahar Gvirtzman, the team combined cutting-edge experiments with innovative theoretical models to demonstrate how slow, aseismic processes precede and enable seismic ruptures. By incorporating the finite width of fault interfaces—a factor often overlooked in traditional models—the researchers showed that localized stress and geometric constraints are key drivers of earthquake initiation.
“Our findings challenge and refine conventional models of rupture dynamics,” said Prof. Fineberg. “We show that slow, aseismic processes are a prerequisite for seismic rupture, driven by localized stress and geometric constraints. This has profound implications for understanding when and how earthquakes begin.”
The study employed high-speed imaging and advanced methodologies to observe the nucleation of frictional motion. The researchers discovered that earthquake initiation begins as small, slow-moving two-dimensional patches of frictional motion. These patches gradually expand and transition into the rapid dynamics described by classical fracture mechanics, marking a significant leap in understanding the process.
Key to the findings is the role of fault geometry in controlling nucleation dynamics. By integrating the finite width of fault interfaces into their models, the researchers refined existing theories and provided fresh insights into the structural and mechanical factors influencing seismic activity. This focus on geometric transitions highlights the importance of seemingly minor precursors, such as slow, aseismic processes, which could hold critical information for predicting earthquakes.
The study’s implications extend beyond earthquake science, offering insights into material strength, fracture dynamics, and the development of predictive models for seismic activity. The newly developed framework also enhances understanding of everyday processes involving friction and material fracture, with potential applications in engineering and disaster mitigation.
“Even seemingly ‘quiet’ seismic precursors, which might previously have been overlooked, could hold critical information about impending seismic events,” explained Prof. Fineberg. “This discovery has the potential to inform predictive models and improve our ability to anticipate and mitigate earthquake risks.”
The research, published in Nature, represents a significant step forward in understanding the mechanics of earthquake ignition and could pave the way for more accurate earthquake prediction and risk assessment.